Access success stories
Ever since the beginning of Laserlab-Europe, one of its most important features has been the Transnational Access Programme. Up to now, about 1,200 scientists from institutions outside Laserlab-Europe had access to Laserlab facilities to perform their experiments. Proposals for Transnational Access are reviewed by an external and independent Access Selection Panel on the basis of scientific merit. Access to Laserlab facilities is free of charge; travel and accommodation expenses of visits with a typical duration of two to six weeks are covered by the Programme.
Each access project has its unique history and benefits. In many cases, the host researchers are not only involved in assisting their guests, but form an integral part of the research project. As a result, the host institutions benefit directly from the programme. Through the years, many long-term research collaborations have been formed as a result of Transnational Access. On the following pages, we highlight a few particularly successful access projects.
Relativistic-intensity 1.5-cycle light waveforms at kHz repetition rate leads to observation of laser waveform effects in laser-plasma accelerator (LOA)
Generating ultrashort laser transients capable of steering relativistic light forces with sub-cycle precision during laser-plasma interactions has been a long-standing challenge, fuelled by the prospect of developing ultrafast particle and radiation beams for applications. In a recent Transnational Access project performed at French Laserlab-Europe partner LOA, an international team made up of researchers from Berlin, Goettingen and Institut Polytechnique de Paris have developed a source of relativistic-intensity near-single-cycle laser pulses and observed the first evidence of laser waveform effects inside a laser-plasma accelerator. These results have been published in Light: Science & Applications.
There is a push in ultrafast science to merge two distinct classes of lasers: those achieving relativistic intensity and those with pulse durations approaching a single light cycle. While the former laser class traditionally involves large-scale amplification chains, the latter class requires extremely precise spatiotemporal control over the laser field. This feature is at the heart of the recent work done by the Laserlab-Europe collaboration on a laser-plasma accelerator (LPA) driven by near-single-cycle laser pulses (Fig. 1). In order to reach relativistic energies, electrons must be injected into the LPA via field ionisation close to the peak of the pulse, which heavily depends on the carrier-envelope phase (CEP) of the pulse as it determines the final electron beam properties (spectrum and charge). However, the injection event must be highly localised in space and time to avoid field effects being washed out during laser propagation, thereby placing stringent demands on laser stability. Developing such a stable few-cycle light source was very challenging. The key to success was the adoption of the stretched flexible hollow fiber technology, pioneered by Tamas Nagy (MBI) and Peter Simon (Laser-Laboratorium Goettingen e.V.), to efficiently post-compress the high-energy pulses produced by the CEP-stable Ti:sapphire chirped pulse amplifier built by the LOA team. This would eventually lead to the generation of multi-mJ energy pulses with sub-2-cycle duration and stable CEP for the first time in 2014 [1]. In 2017, increased energy from the laser amplifier and improved integration of the hollow fibre compressor enabled the LOA team to drive a relativistic electron LPA for the first time at kHz repetition rate [2], albeit without CEP control due to insufficient laser stability.
Figure 1: Schematic top-down view of the electron LPA developed at LOA and snapshot of the 1.5-cycle laser focal spot size
(2.5 x 2.8 μm 2 ) resulting in relativistic driving light intensities (5 x 10 18 W/cm 2 ) [3].
Our latest engineering efforts have now resulted in record pulse duration (1.5 cycle), terawatt peak-power and the pulse stability required to successfully observe field effects inside the LPA (< 0.3 % rms over several hours). Raw electron spectra recorded from the LPA (Fig. 2a) show clear CEP effects although they tend to wash out towards the end of the CEP cycling scan. Nevertheless, the averaged electron spectra for the first two CEP cycles (Fig. 2b) show a correlation for relative CEP changes that clearly outweighs the spectral fluctuations, which naturally occur due to the extreme nonlinearity of the interaction. The next step of the collaboration will focus on enhancing the stability and robustness of the LPA so the electron beam becomes usable for applications downstream.
Figure 2: a) Top: electron spectra dN/dE (arb. units) obtained
while varying the CEP of the driving field (each spectrum was
obtained by averaging of 500 laser shots). Bottom: the measured
CEP (blue dots) and the command CEP values sent to the
feedback loop (black line). b) Averaged spectra for the first (top)
and second (bottom) CEP cycles. The solid dark lines represent
the average spectra, and the light blue areas indicate the
standard deviation due to spectral fluctuations [3].
Rodrigo Lopez-Martens and Stefan Haessler (LOA)
[1] F. Böhle et al., Laser Physics Letters 11: 095401, 2014
[2] D. Guénot et al., Nature Photonics 11: 293, 2017
[3] M. Ouillé et al., Light: Science & Applications 9:47, 2020
Creating 3D images with X-ray flashes using computer vision (LIDYL)
Lensless microscopy with X-rays, or coherent diffractive imaging, is a promising approach in imaging. While two-dimensional images can already be created quickly and efficiently, 3D images are still a challenge. In a recent Transnational Access project performed at French Laserlab partner LIDYL, an international team made up of researchers from Hannover, Hamburg, Lisbon and Paris-Saclay has now demonstrated a new imaging technique that enables reconstruction of a three-dimensional image based on a single acquisition, by combining computer vision and lensless imaging algorithms. The results have been published in Nature Photonics.
At a nanometre scale, the ability to gain insights into the 3D properties of artificial or biological systems is often critical. This information is, however, difficult to retrieve as most techniques provide only two-dimensional projections along the imaging axis. Nowadays, intense ultrashort XUV and X-ray pulses allow realising nanometre scale stud-ies and ultrafast time-resolved 2D movies. Unfortunately, these existing methods are not easily extended to single shot 3D images. As a rule, a three-dimensional image of an object is generated mathematically from hundreds of individual images. This means a high expenditure of time, large amounts of data and high radiation sums.
In the framework of the FET Open project VOXEL, we have now succeeded in significantly accelerating this pro-cess by developing a method in which two images of an object can be taken with a single ultrafast acquisition from two different viewing directions, which are then combined to form a spatial image - similar to how the human brain forms a stereo image from the two slightly different imag-es of the two eyes. The method of computer-aided spatial vision is already established in the field of machine vision and robotics. Here it is used for the first time in the field of imaging with X-rays.
This first demonstration uses two XUV coherent beams based on high harmonic generation which are focused on a 3D nanoscale sample. To generate the two subbeams, we insert a grazing incidence prism between the off-axis parabola and the sample. Two silicon mirrors are adjusted such that the two beam foci overlap on the sample, with a controllable angle. Moreover, the setup enables finetuning of the time delay between the two pulses, and can alter-natively be used to perform femtosecond time-resolved X-ray-pump/X-ray-probe experiments.
The two beams are diffracted by the sample and the far field patterns are recorded simultaneously on a single X-ray CCD camera. A typical set of stereo diffraction patterns set at an angle of 19° is shown in Fig. 1. They are slightly dif-ferent, which reflects the different observation angles. The slight overlap between the two diffraction patterns does not affect the data. Indeed, in our case, the maximal useful diffraction angle is limited by the signal-to-noise ratio. The number of useful diffracted photons on each diffraction pattern is roughly equivalent (few 107 photons per shot). Each diffraction pattern is isolated and inverted independently using a HIO “difference map” algorithm.
Figure 1: Dual diffraction patterns, recorded simultaneously on a single XUV CCD (the CCD image is rotated by 90°). The left (right) diffraction pattern corresponds to the beam coming on the sample from the top (bottom). The diffraction pattern extends well with a slight overlap at high frequency that can be numerically corrected. This effect could be circumvented by increasing the stereo angle and using an arrangement with two adjacent CCD cameras or a large area PN-CCD detector.
Figure 2: 2D amplitude reconstructions of the sample from the two stereo views. (a) and (b) show the reconstructions corresponding to the top and bottom views of the sample. They are obtained as the coherent averages of, respectively, 45 best reconstructions from independent runs of the CDI algorithm. Each view reaches a spatial resolution of 90 nm which allows to observe details of our nanoscale sample. For comparison, we present In the top-right corner the SEM (scanning electron microscopy) image of the sample observed at a 45° angle.
Figs. 2(a) and (b) show the amplitude reconstructions of, respectively, the bottom and top diffraction patterns, corresponding to the top and bottom views of the sample, as the average of several independent runs of the phase retrieval algorithm. The spatial resolution of each view is measured to be 130 nm. Differences between the two views are clear: the positions of the opaque cross inside the gap vary. No gap is visible between the lid and the hole at the bottom left and top right parts of Fig. 2(a).
The method allows gaining qualitative 2D structural and spatial information from two observation angles in one acquisition. However, it is possible to go further and gather some quantitative depth information from those images. Indeed, from the pair of reconstructed views of the sample one can compute the disparity map. Disparity refers to the distance between two corresponding points in the two images of a stereo pair. By matching each pixel from one image to the other, one can calculate the distance between them to produce an image. We attribute then the disparity value for that pixel to the corresponding image. The disparity map can, then, be converted into depth information by a simple equation given the geometry of our setup:
In equation (1), z is the relative depth value of the point P of the object, d(P) is P’s disparity value and Φ1 , Φ2 are the angles between the plane perpendicular to the CCD and each stereo beam, respectively. From eq. (1) one can notice that the resolution on the depth axis increases with the angle between the two illuminating beams. However, there is an upper limit for this angle which depends on the sample’s structure, defined by a minimal presence of identical features in both views, mandatory in order to be able to calculate the disparity values.
Figure 3: Disparity maps and 3D reconstructions of the sample. (a), (b), (c): Disparity maps obtained from the correspondence between the right and left views, in this order – bottom and top for the experimental case. The colour scale is defined in pixels. (d), (e), (f ): 3D reconstruc-tions of the sample. While (a) and (d) correspond to a numerical simulation performed with an amplitude 3D sample in the experimental geometry, (b) and (e) show the same results assuming a phase sample – different refraction index materials for the cross and the membrane with a cross-shaped cut. (c) and (f ) present the experimental disparity map and perspective reconstruc-tion from the images shown in Fig. 3(c) and (d). Note that the colour scale represents the depth value z, for better visualization.
Figures 3(a), (d) and (b), (e) show the results of simula-tions. (a) and (b) present the disparity maps, while (d) and (e) show snapshots of the 3D reconstructions. On the disparity map of Fig. 3(a), which corresponds to a pure amplitude sample, some information is missing due to the lack of common details visible in both views. This brings on evident artefacts in the reconstruction (Fig. 3(d)), where the lid is linked to the membrane.
In Fig. 3(b) and (e), on the other hand, the simulation was realised with a phase sample. Having two stereo views with information on the phase shifts unveils the existence of superimposed planes, which makes possible the retrieval of disparity values on structures which were before hidden behind the membrane. This allows a 3D rendering with fewer artefacts and more details on the structure of the sample. Figs. 3(c) and (f ) show the experimental results from the image reconstructions of Figs. 2(a) and (b). While the cross shape of the hole in the membrane is clearly visible, the reduced signal quality makes the reconstruction of the 3D lid shape more difficult. Note that the cross presents the same non-real connections with the membrane as in the simulation of the pure amplitude sample. Our geometry leads to a voxel size of 96x96x146 nm3. However, due to the reduced quality of the reconstruction, the actual depth resolution cannot be estimated.
The method described above can be designed for any coherent X-ray beamline and applications are foreseen in single-shot 3D structural imaging of high-impact systems, ranging from biological samples to ultrafast physical processes. A demonstration using data from a soft X-ray synchrotron experiment is also performed and is available in the Nature Photonics paper (see reference below).
We expect that the process will have a major influence on the 3D structure imaging of individual macromolecules and see potential applications in biology, medicine and industry. Other machine learning algorithms are expected to have a huge potential in those fields. For example, the protein structure of a virus, which has a crucial influence on the function and behaviour of a virus and plays a decisive role in medical diagnoses, could be investigated quickly and with little effort in the future by using face recognition type algorithms.
Hamed Merdji (LIDYL)
J. Duarte et al., Nature Photonics 13: 449-453, 2019
Micro-LIBS imaging of mollusc shells: a window to the past (IESL-FORTH)
In a recent article in Scientific Reports [1] , resulting from a Laserlab-Europe transnational access project, scientists at IESL-FORTH, collaborating with the Max Planck Institute for the Science of Human History and the School of Geography, University of Melbourne, have shown that LIBS microscopy enables extensive elemental mapping of mollusc shells, providing an insight into the rich environmental archives contained in such specimens. This is a major step forward for the exploitation of mollusc archives to study climate in a range of applications, including ocean circulation, glacial/interglacial climates, and anthropogenic climate change.
Shellfish have played a significant role in the diet of prehistoric coastal populations, providing valuable nutrients. They are a common find at archaeological sites all over the world, usually in huge numbers, and researchers have long explored how they could be used to make inferences about the environments that humans experienced at those locations in the past. However, although techniques have been developed to extract valuable climate-related information from shells, it has been too expensive to obtain data on a scale beyond individual and isolated records. The current study presents a technique to use rapid laser imaging to increase the number of analysed shell records to previously unknown scales, and thereby greatly expand the time periods and accuracy of the reconstructed records.
The effort was led by Niklas Hausmann at IESL-FORTH in the framework of his Marie Skłodowska Curie fellowship project ACCELERATE . He joined the research group of Demetrios Anglos (University of Crete and IESL-FORTH) and collaborated with Panagiotis Siozos, senior researcher, and Andreas Lemonis, automation and programming expert, developing an automated scanning micro-LIBS (laser-induced breakdown spectroscopy) apparatus that enabled mapping of large numbers of shells of the course of the project. It is important to note that this research emerged from a Laserlab-Europe access project.
Map of sample locations that were analysed in the Mediterranean study.
It was in December 2013 that Hausmann, then a PhD student at the University of York, UK, approached Costas Fotakis and Demetrios Anglos at the Ultraviolet Laser Facility-FORTH inquiring on the possibility to use their laser for carrying out oxygen isotope analysis on shells. After an access proposal was approved, Niklas Hausmann visited ULF-FORTH and carried out a series of exploratory experiments in April-May 2014.
Following two weeks of hard work, Hausmann and his co-workers were able to obtain the first LIBS images showing variations of the magnesium to calcium (Mg/ Ca) concentration on sections of mollusc shells [2, 3] . These variations, traced along the shell growth lines, were con- sistent with seasonal temperature variations, showing the potential of micro-LIBS as a new tool for obtaining high- resolution elemental images of shell surfaces.
This success was enough to spark a more consistent ef- fort to investigate LIBS mapping in detail, and led to the ACCELERATE project. Within this project, mollusc shells from a range of countries and archaeological sites were analysed, covering 20 countries on all continents and ranging back into past time periods with shells collected by Neanderthals. Most shells, however, dated to modern periods, where the elemental composition could be compared to readings from instrumental data, as was the case for the study in Scientific Reports[1]. Here LIBS allowed to analyse quickly and at low cost shells from across the Mediterranean in a wide and multiregional study that compared different localities, rather than only one, resolving major concerns in the field regarding the improvement of rapid and low-cost climate proxies such as Mg/Ca ratios in shells.
So far, it had been hard to link such elemental ratios of shell carbonate to sea surface temperatures because the interpretation of the measurements was hampered by variations in study location as well as vital or physiological effects between and within individual mollusc specimens. Having identified the Mediterranean limpet Patella caerulea as an ideal species to assess elemental variations through LIBS, elemental maps and line scans of 19 shell specimens from 9 sites were produced for the study.
Records showing the good correlation of expensive oxygen isotope records (above) and rapid LIBS records.
The Mg/Ca ratio maps of almost all specimens show repeating bands of high and low intensity throughout the entire length of growth increments, corresponding to the seasonal changes in sea surface temperature known from satellite measurements. Only two shells – the ones that had grown for less than a year – lacked these bands. As many as 8 out of the 19 specimens, however, showed additional Mg/Ca patterns unrelated to sea surface temperature, and in two of those specimens this prevented reliable temperature reconstruction. Such problematic specimens would skew the sea surface temperature record if measured without being recognised. The observed seasonal changes in Mg/Ca ratios obtained by LIBS allow archaeologists to determine at relatively low costs in which season the specimen has been consumed, which is of importance for the study of past human and animal behaviour.
Hausmann is now seeking funding to set up a dedicated micro-LIBS facility for large-scale mollusc screening, based on the outcomes of the ACCELERATE project, which did not only find new ways of analysing temperature changes, but also to reveal growth structures within shells. These can be key to efficiently plan analyses using other geochemical proxies, such as oxygen isotope analysis or clumped isotope analysis, which are more expensive.
In addition to molluscs, there are other carbonate archives with their own interesting research trajectories that LIBS mapping can address. Stalagmite and corals have previously been shown to provide important elemental records of climate change and would benefit from rapid analysis or screening prior to more detailed geochemical studies. The team at FORTH investigates these potential applications.
Niklas Hausman (University of York), Panagiotis Siozos & Demetrios Anglos (IESL-FORTH)
1. N. Hausmann et al., Scientific Reports 9: 3698, 2019
2. N. Hausmann et al., J. Anal. At. Spectrom. 32: 1467, 2017
3. N. Hausmann et al., pp 725-740, in: N. Rasul, I. Stewart (eds), Geological Setting, Palaeoenvironment and Archaeology of the Red Sea, Springer, Cham, 2019
Measuring the binding energy of molecular hydrogen to test fundamental physics (LaserlLaB Amsterdam)
Seventy years after the development of quantum electrodynamic theory, the simple hydrogen molecule is still at the heart of the most stringent tests of our most successful physical models. A recent Laserlab-Europe transnational access collaboration between the groups of Wim Ubachs (LaserLaB Amsterdam) and Frédéric Merkt (ETH Zürich) led to the most accurate experimental determination so far (one part in a billion) of the binding energy of the hydrogen molecule. The results have been published in Physical Review Letters.
Measuring the binding energy of molecular hydrogen to test fundamental physicsMolecular hydrogen with its four constituents, a pair of protons and a pair of electrons, permits the most accurate first-principles calculations among any neutral molecules. Equally accurate experimental measurements of properties like the binding energy of the hydrogen molecule thus allow us to test the validity of such calculations and their underlying models.
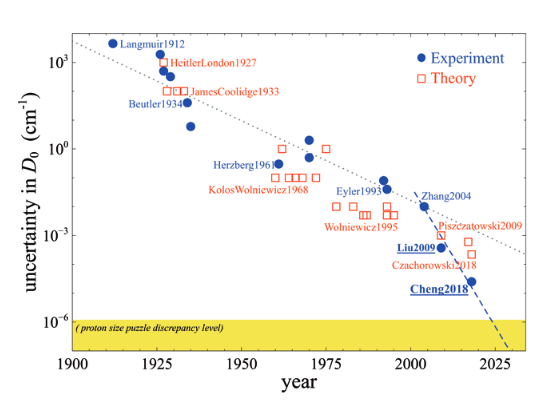
molecule.
Comparison of measurements and calculations can even be used in searches for physics beyond the Stand-ard Model, which is the topic of the ERC Advanced Grant project of Wim Ubachs. The simple idea is that, assuming that we understand quantum electrodynamic theory (QED) completely, a real discrepancy with the measurements must point to new effects that were unaccounted for and may either assert the incompleteness of QED or even the presence of new interactions outside the Standard Model.
Steady improvements in the experimental and theo-retical investigations have resulted in orders of magnitude improvements in accuracy of both measurements and calculations of the binding energy (or, equivalently, dissocia-tion energy) of molecular hydrogen over several decades as presented in Figure 1. The dynamics of this race in precision has mutually motivated innovations in the measurements and calculations, and sets the stage for the present experimental investigation.
The indirect approach adopted by the teams of Wim Ubachs and Frédéric Merkt in this project is based on a “thermodynamic cycle”, which involves ionisation and dissociation energies of the neutral and ionic molecules as well as the ionisation energy of the atom. As depicted in Figure 2, this looks at first glance more complicated than it should be. In fact, this approach solves severe difficulties encountered in the previous approach pioneered by Herzberg, which involved an extrapolation to the onset of the dissociation continuum.
The price to pay of the present approach is performing a sequence of spectroscopic experiments that link the mo-lecular electronic ground state to an intermediate excited state; and further connecting this intermediate state to the ionisation limit of the molecule, which includes a sophisti-cated quantum defect extrapolation. In addition, the dissociation energy of the H2+ ion obtained from accurate calculations, and the ionisation limit of the H atom extracted from accurate measurements are needed. Surprisingly, this enables the most accurate determination (1 part in 109) of any molecular bond strength.
While there has been a long scientific association between the groups of Wim Ubachs and Frédéric Merkt, the present collaboration is a natural follow-up of an earlier campaign to measure the dissociation energy of hydrogen, launched some ten years ago. Back in 2008, during dinner high up in the Swiss Alpine peak of Diavolezza after an intense day of science and ski, it was decided to perform the last link of the experimental chain at ETH Zürich. (The energy difference between the ground and intermediate EF state had already been measured in Amsterdam.) The combined experimental efforts eventually led to more than order of magnitude improvement on all previous determinations.
At the time, the experimentalists thought that it would take several years if not decades for the theorists to catch up. Such complacency was shattered quickly, when Krzysztof Pachucki and co-workers announced impressive improvements in their calculations just a few months later.

That the improved measurement presently described took almost ten years after the previous investigations is due to the required developments in pursuing a slightly different path, that is using the so-called GK state instead of the EF state as an intermediate level. This seemingly slight adaptation required substantial changes in the spectroscopic investigations both at Amsterdam and Zürich.
A key ingredient for the LaserLaB Amsterdam experiment is a special nonlinear crystal, KBBF, which uniquely enables frequency doubling in the vacuum ultraviolet range. To acquire such a crystal, which is only produced in China, Wim Ubachs visited Shuiming Hu in Hefei where he proposed a collaboration. This turned out to be quite a challenge, as the Chinese government has strictly restricted access of this crystal outside the country. When the KBBF crystal finally arrived in Amsterdam, it had taken more than a year of efforts and negotiations, before eventually receiving the approval of the Chinese Academy of Sciences, the very tip of their academic pyramid.
The in-house developed narrowband titanium sapphire-based pulsed source at LaserLaB Amsterdam offers extreme frequency resolution (Q-factor ~1x108) while providing broad wavelength tunability in the infrared range ~700 -850 nm. The design is based on a cw-seeded master oscillator cavity with a 532-nm pulsed pump, with the oscillator output pulse further amplified in multi-pass configuration in another Ti:Sa crystal. (Radiant Dyes Gmbh, has been granted licence to commercialize this system.)
In addition, the Ti:Sa cw-seed frequency is locked to an optical frequency comb that is referenced to a Cs atomic clock in Kjeld Eikema’s ultrafast lab, also at LaserLaB Amsterdam, to obtain the highest accuracy in frequency calibration. With an IR output of several tens of mJ energy per pulse, subsequent second harmonic generations using both a BBO crystal and the mentioned KBBF crystal pro-duced radiation at 179 nm.
By performing Doppler-free two-photon excitation of the X ground state to the intermediate GK state in a hydrogen molecular beam, the UV transition frequency is measured at a relative accuracy of 2 parts in 1011. To reach such accuracy, all sorts of systematic effects, including light power shifts, residual Doppler effects, as well as frequency chirp in the laser source had to be measured and compensated. It is noted that this GK-X measurement is the first direct excitation ever and hence it is even more remarkable that such accuracies could be achieved.
The parallel experiment at Zürich provided the link from the intermediate GK state to the molecular ionisation energy. The alternative approach to the GK state enabled the possibility of cw excitation to high-lying Rydberg states, which drastically improved the accuracy. With careful systematic checks, this enabled the determination of this frequency interval to an accuracy that is more than 50 times better than that obtained by the same collaboration in 2009. The combination of all energy intervals (see Figure 2) enabled the determination of the binding or dissociation energy of molecular hydrogen to an accuracy in the level of 1 part per billion.
The present measurement results are in agreement with the most recent calculations, thus validating that the present theory is correct at the level of the combined uncertainty of both measurement and calculations. In fact, this combined uncertainty can be used to constrain the effects of hypothetical fifth forces (in reference to the four known fundamental forces) or even hidden extra dimensions in space.
Such new physical effects are traditionally searched for in huge particle physics experiments such as the Large Hadron Collider (LHC) at CERN with TeV energy scales or even higher-energy phenomena as those occurring in supernovae. In contrast, complementary searches in table-top setups such as those described here rely on probing the most delicate perturbation in the energy levels rather than brute force of particle creation, and greatly leverage upon the unmatched frequency precision that can be achieved in spectroscopy (femto-eV resolution), as well as the extreme accuracy in the theoretical description of simple atomic or molecular systems.
Edcel Salumbides, LaserLaB Amsterdam
C.-F. Cheng et al., Phys. Rev. Lett. 121, 013001 (2018)
Figure 3: The experimental setup used at LaserLaB Amsterdam.